I have written articles for Cutting Tool Engineering for a decade. If you have read any of those stories, you may have recognized that I possess an affinity for old-school machining and engineering. I like machines with handles, cranks and scraped ways. I prefer indicators to controls and enjoy the smell of cutting oil.
Although the art of conventional machining and toolmaking is my preference, my living has been made creating modern machining processes and programming the most advanced machine tools available. Modern technology is the only path to the productivity gains needed to further our economy, so I appreciate the benefits derived from up-to-date machining techniques and machine tools.
My love for the old ways came from working on machine tools from the 1940s and ’50s when I learned the craft. The experiences gained on those old machines allowed me to solve problems encountered on modern machines. A unique symbiotic relationship exists between old-school machining and modern manufacturing that makes me consider how the new and the old will interact in the future.
I see a gap in the way we are educating the next generation of machinists and engineers. Much of our vocational education focuses on CAD, CNC programming and similar technologies. Our foundations seem to be shifting away from conventional machining methods, especially at the university level.
I believe that manufacturers are pushing the educational system toward high-tech manufacturing because of the tremendous shortage of people in the labor market. Manufacturers need employees in front of machining centers. It is difficult to argue that this is the wrong method if you have tried to hire a machinist anytime in the past decade. However, I have seen young machinists and toolmakers struggle significantly at machine shops when managers have thought that any young person with a certificate from a local trade school could walk through the doorway and be effective.
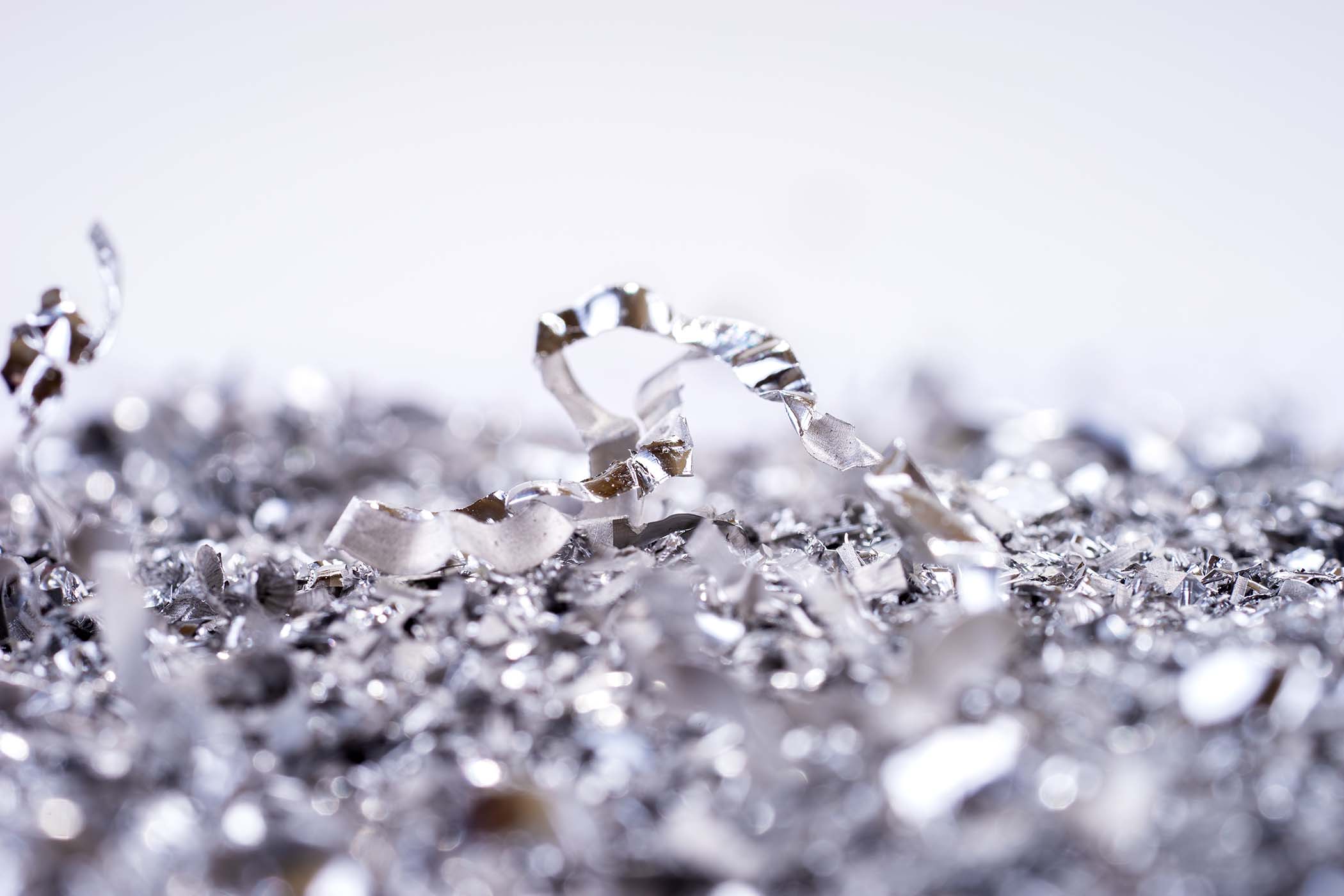
A conventional machinist or toolmaker does more than operate a machine. Machining is a science practiced by artisans. Making chips on a conventional lathe or knee mill trains the senses because machinists use most of their senses when making chips. Sight, sound, smell and touch provide a continuous stream of feedback used to assess the chip-making process. With these sensory inputs, machinists adjust, caress, cajole and manipulate machine tools to deliver the desired results.
Broken tools, scrapped workpieces, pretty chips, good finishes, successes and failures all influence the senses. The only way to train the senses to recognize successes and failures is to stand at a machine and make chips. Isolating a student from these experiences meaningfully affects skill development. Our engineering and machining students are being rushed into CNC work before they see substantial time on the handles and cranks of manual machines.
Distance from conventional machines limits flexibility at a shop. I have had many talented CNC machinists who were unable to cross the floor to a conventional machine because they did not have the skill to use it. At a prior employer, we received large, very expensive steam turbine housing bolts with oversized pitch diameters. Our build schedule forced us to repair them in-house, and we did it on a 40-year-old conventional lathe. Picking up and chasing the thread on a CNC lathe is impossible, so our salvation was two machinists who had learned their craft on Navy ships running old machines.
Conventional machinists also have a better understanding of part geometry and how to manipulate a machine and its setup to generate tricky geometry. I once saw engineers, CNC machinists and mechanics struggle with a vertical machining center that was making off-center holes on a rotary table. The first hole would be good, but the second would be off-center. An old toolmaker heard workers lamenting the situation and the hours they had spent trying to resolve the issue. The toolmaker went to the machine with an ancient indicator and solved the problem within 30 minutes by realigning the collet. Because he had spent years shifting parts off-center on lathes to make eccentric shapes, he easily recognized the plight at hand.
When you can convince them to take the job, good conventional machinists make very good CNC machinists since they readily can identify the onset of failures. Discolored chips, strange sounds, questionable finishes and bad part geometries are early warning signs that conventional machinists have learned to respect after countless hours in front of conventional machines.
Skills learned on conventional machines also have direct application to more obscure processes. For instance, broaching is a common method for producing radial blade slots in large gas turbine discs. Everything about these machines and their parts is expensive, and broaching is usually the last major machining operation on disc forgings that can cost hundreds of thousands of dollars. So problems with broaching can be super-expensive. Just like the example of the rotary table, I watched an experienced conventional machinist fix a serious problem on a $250,000 disc with a $1 shim after engineers spent a week looking at the machine.
We risk losing our ever-shrinking economic advantage to the competition as well. Travel to Europe and Asia, and you will find apprenticeships where students learn the craft in traditional ways. I recently visited a machine tool builder in Germany. Inside the shop was a classroom with about 20 students learning to be machinists. They were squaring blocks of steel armed merely with files. In the United States, this is a fabled activity that only very experienced — that is, old — toolmakers and machinists have completed.
We quickly are losing our skilled machinists and toolmakers to retirement, yet we have not effectively passed their skills to the next generation. Our schools are too focused on building manufacturing technology centers with computer-based equipment and babbling about the Internet of Things because it is the latest fad. Our student machinists and engineers need to stand in front of conventional machines and make chips. Students must be challenged by difficult geometries and deprived of computer-based problem-solving before being introduced to computer-based manufacturing. Sure, we can survive with only CNC machines. But creative, elegant, simple solutions to machining problems will begin to disappear.
Related Glossary Terms
- broaching
broaching
Operation in which a cutter progressively enlarges a slot or hole or shapes a workpiece exterior. Low teeth start the cut, intermediate teeth remove the majority of the material and high teeth finish the task. Broaching can be a one-step operation, as opposed to milling and slotting, which require repeated passes. Typically, however, broaching also involves multiple passes.
- centers
centers
Cone-shaped pins that support a workpiece by one or two ends during machining. The centers fit into holes drilled in the workpiece ends. Centers that turn with the workpiece are called “live” centers; those that do not are called “dead” centers.
- collet
collet
Flexible-sided device that secures a tool or workpiece. Similar in function to a chuck, but can accommodate only a narrow size range. Typically provides greater gripping force and precision than a chuck. See chuck.
- computer numerical control ( CNC)
computer numerical control ( CNC)
Microprocessor-based controller dedicated to a machine tool that permits the creation or modification of parts. Programmed numerical control activates the machine’s servos and spindle drives and controls the various machining operations. See DNC, direct numerical control; NC, numerical control.
- computer-aided design ( CAD)
computer-aided design ( CAD)
Product-design functions performed with the help of computers and special software.
- lathe
lathe
Turning machine capable of sawing, milling, grinding, gear-cutting, drilling, reaming, boring, threading, facing, chamfering, grooving, knurling, spinning, parting, necking, taper-cutting, and cam- and eccentric-cutting, as well as step- and straight-turning. Comes in a variety of forms, ranging from manual to semiautomatic to fully automatic, with major types being engine lathes, turning and contouring lathes, turret lathes and numerical-control lathes. The engine lathe consists of a headstock and spindle, tailstock, bed, carriage (complete with apron) and cross slides. Features include gear- (speed) and feed-selector levers, toolpost, compound rest, lead screw and reversing lead screw, threading dial and rapid-traverse lever. Special lathe types include through-the-spindle, camshaft and crankshaft, brake drum and rotor, spinning and gun-barrel machines. Toolroom and bench lathes are used for precision work; the former for tool-and-die work and similar tasks, the latter for small workpieces (instruments, watches), normally without a power feed. Models are typically designated according to their “swing,” or the largest-diameter workpiece that can be rotated; bed length, or the distance between centers; and horsepower generated. See turning machine.
- machining center
machining center
CNC machine tool capable of drilling, reaming, tapping, milling and boring. Normally comes with an automatic toolchanger. See automatic toolchanger.
- milling machine ( mill)
milling machine ( mill)
Runs endmills and arbor-mounted milling cutters. Features include a head with a spindle that drives the cutters; a column, knee and table that provide motion in the three Cartesian axes; and a base that supports the components and houses the cutting-fluid pump and reservoir. The work is mounted on the table and fed into the rotating cutter or endmill to accomplish the milling steps; vertical milling machines also feed endmills into the work by means of a spindle-mounted quill. Models range from small manual machines to big bed-type and duplex mills. All take one of three basic forms: vertical, horizontal or convertible horizontal/vertical. Vertical machines may be knee-type (the table is mounted on a knee that can be elevated) or bed-type (the table is securely supported and only moves horizontally). In general, horizontal machines are bigger and more powerful, while vertical machines are lighter but more versatile and easier to set up and operate.
- pitch
pitch
1. On a saw blade, the number of teeth per inch. 2. In threading, the number of threads per inch.
- sawing machine ( saw)
sawing machine ( saw)
Machine designed to use a serrated-tooth blade to cut metal or other material. Comes in a wide variety of styles but takes one of four basic forms: hacksaw (a simple, rugged machine that uses a reciprocating motion to part metal or other material); cold or circular saw (powers a circular blade that cuts structural materials); bandsaw (runs an endless band; the two basic types are cutoff and contour band machines, which cut intricate contours and shapes); and abrasive cutoff saw (similar in appearance to the cold saw, but uses an abrasive disc that rotates at high speeds rather than a blade with serrated teeth).